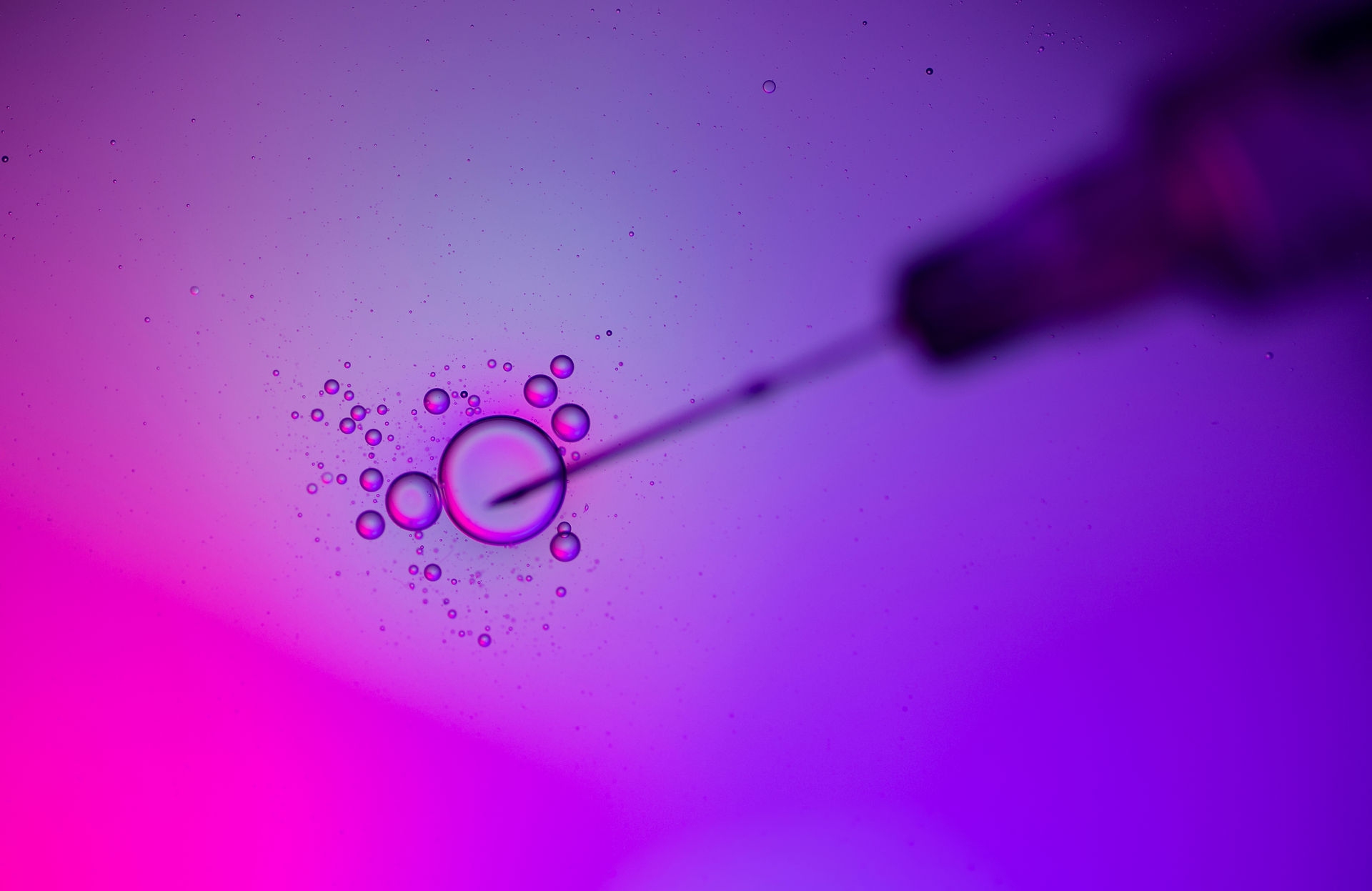
Example - Types of Experiments with LC MS Based Proteomics
Under Construction & Updates - February 2025
We are adding new information on specific topics - weekly . Thank you for your interest!
-
Differential Expression: Quantifies protein changes between conditions (e.g., healthy vs. diseased) to identify differentially expressed proteins, useful for biomarker discovery and drug development.
-
Protein Identification & Characterization: Identifies all proteins in a sample and analyzes post-translational modifications (PTMs) like phosphorylation, crucial for understanding protein function and signaling.
-
Interaction Proteomics: Maps protein-protein interactions using methods like Co-IP or proximity labeling, revealing how proteins work together in complexes and pathways.
-
Targeted Proteomics: Quantifies a pre-selected set of proteins with high sensitivity and reproducibility using SRM/PRM, ideal for biomarker validation and clinical diagnostics.
-
Structural Proteomics: Determines 3D protein structures and interactions using techniques like native MS and cross-linking MS, providing insights into protein function and crucial for drug discovery.
Example - Types of Experiments with LC MS Based Proteomics
Information on Mechanism of Action and Pathway Analysis
Proteomic Mechanism of Action (MOA) Analysis:
-
What it is: Proteomic MOA analysis aims to understand how a drug, treatment, or other perturbation affects biological systems at the protein level. It goes beyond simply observing changes in protein abundance to deciphering the functional consequences of those changes and how they contribute to the overall biological effect.
-
Key Aspects:
-
Identifying Target Proteins: Determining which proteins are directly or indirectly affected by the perturbation. This can involve differential expression analysis, interaction proteomics, or targeted proteomics.
-
Characterizing Post-translational Modifications (PTMs): Analyzing changes in PTMs (phosphorylation, glycosylation, etc.) to understand how protein activity and function are modulated.
-
Mapping Protein-Protein Interactions: Investigating how the perturbation alters protein-protein interactions and complex formation, which can reveal changes in signaling pathways and cellular processes.
-
Analyzing Protein Turnover: Studying changes in protein synthesis and degradation rates to understand how protein levels are regulated over time.
-
Integrating with Other Omics Data: Combining proteomic data with genomics, transcriptomics, and metabolomics data to gain a more comprehensive understanding of the MOA.
-
-
Applications:
-
Drug Development: Understanding the MOA of a drug is crucial for optimizing its efficacy and minimizing side effects. Proteomics can help identify drug targets, predict drug response, and discover biomarkers for patient stratification.
-
Toxicology: Proteomics can be used to study the MOA of toxic substances and identify potential adverse effects.
-
Systems Biology: Proteomic MOA analysis contributes to a systems-level understanding of biological processes and how they are affected by perturbations.
-
-
Pathway Analysis:
-
What it is: Pathway analysis is a computational approach used to interpret proteomic (and other omics) data in the context of biological pathways and networks. It helps to understand the functional implications of changes in protein expression or PTMs by mapping them onto known pathways and identifying enriched or dysregulated pathways.
-
-
Key Aspects:
-
Pathway Databases: Utilizing databases like KEGG, Reactome, and WikiPathways, which contain information about known biological pathways and protein-protein interactions.
-
Enrichment Analysis: Determining whether a set of proteins or PTMs is significantly enriched in a particular pathway. This can be done using statistical methods like hypergeometric test or Fisher's exact test.
-
Network Analysis: Constructing and analyzing protein-protein interaction networks to identify key regulatory proteins and pathways.
-
Visualization: Creating pathway diagrams to visualize changes in protein expression or PTMs in the context of biological pathways.
-
-
Tools and Software:
-
Metascape: A web-based tool for gene and protein annotation and pathway analysis.
-
STRING-db: A database of known and predicted protein-protein interactions.
-
Cytoscape: A software platform for visualizing and analyzing biological networks.
-
ReactomePA (R package): For pathway enrichment analysis.
-
ClusterProfiler (R package): For gene ontology and pathway enrichment analysis.
-
-
Integration of MOA and Pathway Analysis:
-
Pathway analysis is a powerful tool for interpreting proteomic MOA data. By mapping changes in protein expression and PTMs onto biological pathways, researchers can gain insights into the specific pathways that are affected by a drug or treatment. This helps to connect the molecular changes observed in proteomics data to higher-level biological processes and ultimately to the overall phenotype. For example, if a drug inhibits a kinase, pathway analysis might reveal that this inhibition leads to downregulation of a specific signaling pathway involved in cell proliferation.
-
-
Challenges and Considerations:
-
Pathway databases are not complete and are constantly being updated.
-
Pathway analysis results should be interpreted cautiously and validated experimentally.
-
Integrating proteomic data with other omics data can be complex but can provide a more comprehensive understanding of the MOA.
-
Emerging Technologies, Applications, and Workflows - Single Cell Proteomics
What is Single-Cell Proteomics?
-
Definition: Single-cell proteomics (SCP) is a field of study that aims to analyze the proteins present within individual cells. This is in contrast to traditional proteomics, which analyzes bulk samples of cells, providing only an average protein expression profile.
-
Significance: SCP allows researchers to understand the heterogeneity of cell populations, identify rare cell types, and study how protein expression varies across individual cells. This has important implications for understanding disease mechanisms, drug responses, and cellular differentiation.
Applications of Single-Cell Proteomics
-
Understanding cellular heterogeneity: SCP can be used to identify different cell types within a population and study how protein expression varies across these cell types.
-
Identifying rare cell types: SCP can be used to identify and characterize rare cell types that may be missed in bulk proteomics experiments.
-
Studying disease mechanisms: SCP can be used to study how protein expression changes in individual cells during disease development and progression.
-
Developing new therapies: SCP can be used to identify new drug targets and develop personalized therapies based on the protein expression profiles of individual cells.
Challenges and Opportunities in Single-Cell Proteomics
-
Sensitivity: One of the main challenges in SCP is the limited amount of protein in a single cell. This makes it difficult to detect and quantify low-abundance proteins.
-
Throughput: Another challenge is the low throughput of current SCP methods. This makes it difficult to analyze large numbers of single cells.
-
Data analysis: The data generated by SCP experiments can be complex and difficult to analyze. New computational tools are needed to analyze these data effectively.
-
Opportunities: Despite these challenges, SCP is a rapidly developing field with great potential. New technologies are being developed to improve the sensitivity, throughput, and data analysis of SCP methods.
As an Emerging Field for Proteomics, here are some references for more information:
- Scaling up single-cell proteomics: https://pubmed.ncbi.nlm.nih.gov/34808355/
-
Single-cell protein analysis by mass spectrometry: https://www.ncbi.nlm.nih.gov/pmc/articles/PMC7767890/
-
Single cell protein analysis for systems biology: https://pubmed.ncbi.nlm.nih.gov/35199955/
20+ years of phospho- proteomics
What is Phosphorylation?
-
Post-translational modification: Phosphorylation is a common type of post-translational modification (PTM), which means it's a chemical change that occurs to a protein after it has been synthesized.
-
Phosphate group addition: It involves the addition of a phosphate group (PO43-) to specific amino acid residues (serine, threonine, or tyrosine) within a protein.
-
Enzymatic regulation: This process is typically catalyzed by enzymes called kinases, while other enzymes called phosphatases remove phosphate groups.
Why is Phosphoproteomics Important?
-
Cellular regulation: Protein phosphorylation is a crucial and reversible modification that plays a central role in regulating numerous cellular processes, including:
-
Signal transduction: How cells communicate and respond to stimuli.
-
Cell cycle progression: The series of events that lead to cell division.
-
Gene expression: The process by which cells read out the information in their DNA.
-
Metabolism: The chemical processes that maintain life.
-
Protein-protein interactions: How proteins interact with each other.
-
-
Disease relevance: Dysregulation of protein phosphorylation is implicated in various diseases, such as cancer, diabetes, and neurological disorders.
-
Drug targets: Many successful drugs target kinases, highlighting the importance of phosphorylation in therapeutic interventions.
How is Phosphoproteomics Performed?
-
Sample preparation: Isolate proteins from your biological sample (cells, tissues, etc.) and digest them into peptides.
-
Phosphopeptide enrichment: Enrich phosphopeptides from the complex peptide mixture using methods like:
-
Metal Oxide Affinity Chromatography (MOAC)
-
Immobilized Metal Affinity Chromatography (IMAC)
-
Strong Cation Exchange (SCX) Chromatography
-
-
Liquid chromatography (LC) separation: Separate the enriched phosphopeptides using reversed-phase liquid chromatography.
-
Mass spectrometry (MS) analysis: Analyze the separated peptides using mass spectrometry to identify and quantify them.
-
Data analysis: Search the acquired MS/MS spectra against protein databases to identify the corresponding peptides and phosphorylation sites.
Applications of Phosphoproteomics
-
Understanding signaling pathways: Map out how signaling pathways are activated and regulated.
-
Identifying disease mechanisms: Investigate how changes in phosphorylation contribute to disease development.
-
Discovering drug targets: Identify potential targets for new therapies.
-
Developing biomarkers: Find potential markers for disease diagnosis or prognosis.
Challenges in Phosphoproteomics
-
Low abundance: Phosphorylated proteins are often present at low levels in cells.
-
Dynamic nature: Phosphorylation is a dynamic process, making it challenging to capture the true phosphorylation state of a protein.
-
Technical complexity: Phosphoproteomics experiments require specialized techniques and expertise.
Example Phosphoproteomics Experiment Considerations:
1. Sample Preparation
-
Protein Extraction: Isolate proteins from your biological sample (cells, tissues, etc.) using appropriate lysis buffers and protease inhibitors to prevent protein degradation and dephosphorylation.
-
Protein Quantification: Determine the protein concentration of your sample using a method like Bradford assay or BCA assay.
-
Protein Reduction and Alkylation:
-
Reduce disulfide bonds in proteins by adding a reducing agent like dithiothreitol (DTT).
-
Alkylate cysteine residues with iodoacetamide (IAA) to prevent disulfide bond reformation.
-
-
Protein Digestion: Digest proteins into peptides using a protease like trypsin, which cleaves peptide bonds at specific amino acid residues (typically lysine and arginine).
2. Phosphopeptide Enrichment
-
Phosphopeptide Enrichment: Enrich phosphopeptides from the complex peptide mixture using one of the following methods:
-
Metal Oxide Affinity Chromatography (MOAC): Uses metal oxides like titanium dioxide (TiO2) or zirconium dioxide (ZrO2) to selectively bind phosphopeptides.
-
Immobilized Metal Affinity Chromatography (IMAC): Uses metal ions like iron (Fe3+) immobilized on a matrix to capture phosphopeptides.
-
Strong Cation Exchange (SCX) Chromatography: Separates peptides based on their charge, allowing for the isolation of phosphopeptides.
-
3. Liquid Chromatography (LC) Separation
-
LC Separation: Separate the enriched phosphopeptides using reversed-phase liquid chromatography (RP-LC). This involves:
-
High-performance liquid chromatography (HPLC) or ultra-high-performance liquid chromatography (UHPLC): Utilize a chromatography system to pump the peptide mixture through a column packed with hydrophobic material.
-
Gradient Elution: Apply a gradient of increasing organic solvent (e.g., acetonitrile) to elute peptides from the column based on their hydrophobicity.
-
4. Mass Spectrometry (MS) Analysis:
-
Analyze the separated peptides using a mass spectrometer. This typically involves:
-
Electrospray Ionization (ESI): Ionize the peptides as they elute from the LC column.
-
Tandem Mass Spectrometry (MS/MS): Fragment the selected peptides to obtain structural information and identify the phosphorylation sites.
-
5. Data Analysis
-
Database Searching: Search the acquired MS/MS spectra against protein databases to identify the corresponding peptides and phosphorylation sites.
-
Phosphorylation Site Localization: Determine the specific amino acid residues that are phosphorylated based on the MS/MS fragmentation patterns.
-
Data Interpretation: Analyze the identified phosphopeptides and phosphorylation sites to understand their biological roles and significance.
Additional Considerations
-
Quantitative Phosphoproteomics: If you want to compare the levels of phosphorylation between different samples, you can incorporate stable isotope labeling (e.g., SILAC) or isobaric tagging (e.g., TMT) into your experiment.
-
Mass Spectrometry Settings: Optimize the mass spectrometry settings (e.g., resolution, fragmentation methods) to achieve the best sensitivity and accuracy for phosphopeptide identification.
-
Data Analysis Software: Use specialized software packages for phosphoproteomics data analysis, as they often have specific algorithms and tools for identifying and quantifying phosphopeptides.
Protein Isoforms - Developing Workflows for Improved Proteome Resolution
What are Protein Isoforms?
-
Variations of a protein: Protein isoforms are different versions of the same protein that arise from a single gene.
-
Alternative splicing: They are often generated through alternative splicing of the pre-mRNA transcript, where different combinations of exons are included or excluded in the mature mRNA.
-
Post-translational modifications: Isoforms can also result from post-translational modifications (PTMs), such as phosphorylation, glycosylation, or ubiquitination, which add chemical groups to the protein.
-
Functional diversity: Isoforms can have distinct functions, localizations, or interactions within a cell, contributing to the complexity and adaptability of biological systems.
Why Measure Protein Isoforms?
-
Understanding biological complexity: Isoforms can play unique roles in cellular processes, development, and disease. Measuring them provides a more complete picture of biological systems.
-
Disease relevance: Changes in isoform expression or distribution can be associated with various diseases, making them potential biomarkers or therapeutic targets.
-
Drug development: Isoform-specific drugs may offer more precise and effective treatments with fewer side effects.
-
Personalized medicine: Isoform profiles could help tailor treatments to individual patients based on their specific disease characteristics.
Methods for Protein Isoform Measurement with LC-MS
-
Bottom-up proteomics:
-
Enzymatic digestion: Proteins are digested into peptides using enzymes like trypsin.
-
LC-MS/MS: Peptides are separated by liquid chromatography (LC) and analyzed by tandem mass spectrometry (MS/MS) to identify and quantify them.
-
Isoform inference: Isoforms are inferred based on the identified peptides, considering factors like unique peptides, shared peptides, and protein sequence databases.
-
-
Top-down proteomics:
-
Intact protein analysis: Intact proteins are analyzed without digestion, preserving isoform-specific modifications and information.
-
LC-MS/MS: Intact proteins are separated by LC and analyzed by MS/MS to determine their mass, sequence, and PTMs.
-
Isoform identification: Isoforms are identified based on their intact mass and fragmentation patterns.
-
-
Targeted proteomics:
-
Selected reaction monitoring (SRM) or parallel reaction monitoring (PRM): These methods target specific peptides or proteins of interest for highly sensitive and quantitative measurements.
-
Isoform-specific assays: SRM/PRM assays can be designed to distinguish and quantify specific isoforms based on unique peptides or transitions.
-
Challenges and Considerations
-
Complexity of proteomes: The presence of numerous isoforms with similar sequences can make it challenging to identify and quantify them accurately.
-
Dynamic range: Isoforms can vary significantly in abundance, requiring methods with high sensitivity and dynamic range.
-
Data analysis: Analyzing complex LC-MS data and assigning peptides to specific isoforms can be computationally intensive.
-
Standardization: There is a need for standardized protocols and data analysis tools to ensure comparability and reproducibility of isoform measurements.
Emerging Technologies and Approaches
-
High-resolution mass spectrometry: Instruments with higher resolution can improve the accuracy of isoform identification and quantification.
-
Machine learning: Machine learning algorithms can be used to analyze complex LC-MS data and improve isoform identification and quantification.
-
Integration with other omics data: Combining proteomics data with transcriptomics and genomics data can provide a more comprehensive view of isoform expression and regulation.
In summary, measuring protein isoforms with LC-MS is a powerful approach to unravel the complexity of proteomes and gain insights into biological processes, disease mechanisms, and potential therapeutic targets. Advancements in LC-MS technology, data analysis, and integration with other omics data are continuously improving the accuracy and comprehensiveness of isoform measurements.
Example - Example Base Protocols for nLC MS Proteomics Experiments
Example - Example Base Protocols for Blood/Plasma Prep
-
Blood Sample Preparation (for Proteomics)
-
Goal: To isolate proteins from blood, remove highly abundant proteins (like albumin and immunoglobulins), and prepare them for MS analysis.
-
Steps:
-
Collection: Collect blood in tubes containing an anticoagulant (e.g., EDTA).
-
Plasma/Serum Preparation: Centrifuge to separate plasma (containing clotting factors) or serum (without clotting factors) from red blood cells. Serum is often preferred as it avoids potential interference from clotting factors.
-
Depletion of Highly Abundant Proteins: Use immunoaffinity depletion columns or kits to remove albumin and other abundant proteins. This significantly improves the dynamic range of detection. Multiple vendors supply these kits.
-
Protein Quantification: Determine protein concentration using a method like Bradford or BCA assay. This is crucial for consistent and comparable results.
-
Protein Digestion (Trypsin):
-
Reduction: Reduce disulfide bonds using DTT (dithiothreitol).
-
Alkylation: Block reduced cysteines with iodoacetamide (IAA) to prevent reformation of disulfide bonds.
-
Digestion: Digest proteins into peptides using trypsin (a protease that cleaves at lysine and arginine residues). This is often done overnight.
-
-
-
Desalting: Remove salts from the peptide mixture using C18 solid-phase extraction (SPE) cartridges or tips. Salts interfere with MS analysis.
-
NanoLC-MS: Peptides are separated by nano-liquid chromatography (nanoLC) and analyzed by tandem mass spectrometry (MS/MS). Common mass spectrometers used in proteomics include Orbitrap, Q-TOF, and triple quadrupole instruments.
Vendor Specific - Protein Quantification: Examples, Perform label-free quantification (LFQ) or stable isotope labeling with amino acids in cell culture (SILAC) quantification using the search engine output. MaxQuant and Proteome Discoverer are commonly used for this.
Vendor Specific - Statistical Analysis: Examples, Use software like Perseus (Max Planck Institute) or other statistical packages (R, Python) to perform differential expression analysis, pathway analysis, and other downstream analyses.
Example - Example Base Protocols for nLC MS Proteomics Experiments
Example - Example Base Protocols for Liver Tissue Prep
-
Liver Tissue Sample Preparation (for Proteomics)
-
Goal: Extract proteins from liver tissue, remove interfering substances, and prepare for MS.
-
Steps:
-
Tissue Collection: Collect liver tissue samples and snap-freeze them in liquid nitrogen to preserve the proteome.
-
Homogenization: Lyse the tissue using a homogenizer in a buffer suitable for protein extraction (e.g., containing protease inhibitors). Mechanical disruption (e.g., bead beating or sonication) can also be used.
-
Centrifugation: Remove cell debris and other insoluble material by centrifugation.
-
Protein Precipitation: Precipitate proteins using trichloroacetic acid (TCA) or other precipitation methods. This helps concentrate the proteins and remove some contaminants.
-
Resuspension and Buffer Exchange: Resuspend the protein pellet in a buffer compatible with trypsin digestion. Buffer exchange can be performed using ultrafiltration/diafiltration or spin columns.
-
Protein Quantification: Determine protein concentration.
-
Protein Digestion (Trypsin): Follow the same reduction, alkylation, and trypsin digestion steps as for blood samples.
-
Desalting: Remove salts.
-
NanoLC-MS: Perform nanoLC-MS analysis.
-
Vendor Specific - Protein Quantification: Examples, Perform label-free quantification (LFQ) or other relative protein quantification methods.
Vendor Specific - Statistical Analysis: Examples, Use software like Perseus (Max Planck Institute) or other statistical packages (R, Python) to perform differential expression analysis, pathway analysis, and other downstream analyses.
Example - Example Base Protocols for nLC MS Proteomics Experiments
Example - Example Base Protocols for Cell Cultures
-
Cell Culture Sample Preparation (for Proteomics)
-
Goal: Isolate proteins from cultured cells and prepare them for MS.
-
Steps:
-
Cell Lysis: Lyse cells using a lysis buffer (often containing detergents like SDS or NP-40) and sonication or other mechanical disruption.
-
Protein Quantification: Determine protein concentration.
-
Protein Digestion (Trypsin): Perform reduction, alkylation, and trypsin digestion. For SILAC experiments, cells are grown in media containing heavy isotopes of amino acids.
-
Desalting: Remove salts.
-
NanoLC-MS: Perform nanoLC-MS.
-
Informatics Considerations: The bioinformatics pipeline is the same as for blood and tissue samples. SILAC experiments require specialized software to analyze the isotope-labeled peptides.
-
- Cell Collection: Harvest cells by trypsinization (for adherent cells) or centrifugation (for suspension cells).
-
Key Considerations:
-
Protease Inhibitors: Always use protease inhibitors throughout the sample preparation process to prevent protein degradation.
-
Phosphatase Inhibitors: If studying phosphorylation, include phosphatase inhibitors.
-
Optimization: Each sample type may require some optimization of the protocol.
-
Experimental Design: A well-designed experiment is crucial for meaningful results. Consider
-
Vendor Specific - Protein Quantification: Examples, Perform label-free quantification (LFQ) or stable isotope labeling with amino acids in cell culture (SILAC) quantification using the search engine output. MaxQuant and Proteome Discoverer are commonly used for this.
Vendor Specific - Statistical Analysis: Examples, Use software like Perseus (Max Planck Institute) or other statistical packages (R, Python) to perform differential expression analysis, pathway analysis, and other downstream analyses.