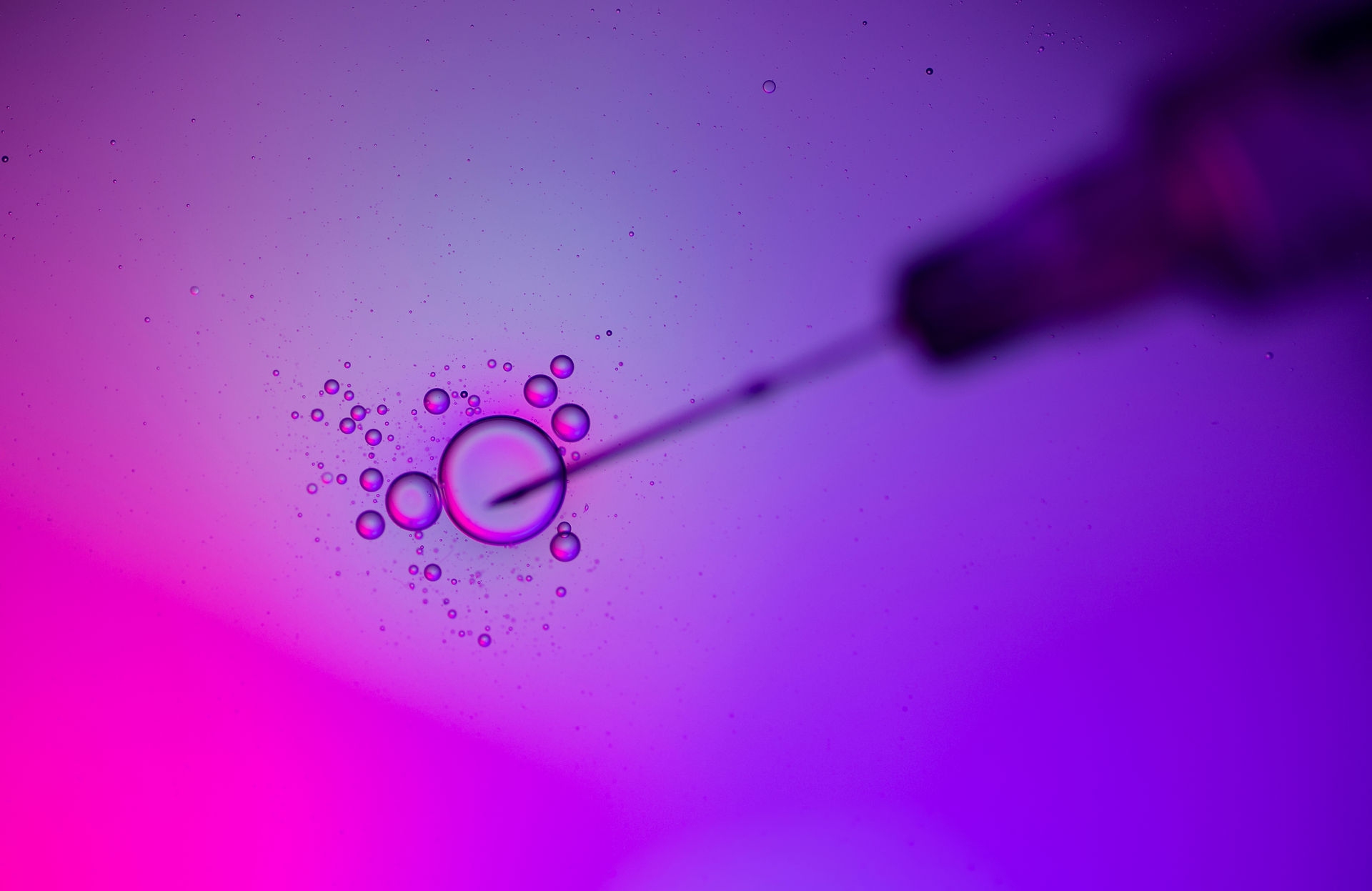
Antibody and Therapeutic Protein Analysis
Under Construction & Updates - February 2025
We are adding new information on specific topics - weekly . Thank you for your interest!
What are Therapeutic Proteins? Therapeutic proteins are complex molecules designed to treat diseases. They include a variety of forms such as:
-
Monoclonal antibodies: Used in immunotherapy and targeted therapies.
-
Hormones: Insulin, growth hormone, etc.
-
Enzymes: Used to treat enzyme deficiencies.
-
Cytokines: Used to stimulate immune responses.
-
Fusion proteins: Combine different protein domains for enhanced therapeutic effect.
Why is Analysis Important?
-
Safety: Ensuring the protein is free from contaminants and has the correct structure to avoid adverse reactions.
-
Efficacy: Confirming the protein has the desired biological activity and potency.
-
Quality: Maintaining consistency in the manufacturing process and product stability.
-
Regulatory compliance: Meeting the requirements of health authorities like the FDA.
Key Analytical Techniques
Therapeutic protein analysis employs a variety of methods:
-
Mass spectrometry (MS): Identifies and quantifies proteins, including post-translational modifications.
-
Chromatography: Separates proteins based on size, charge, or hydrophobicity.
-
Electrophoresis: Separates proteins based on their movement in an electric field.
-
Spectroscopy: Measures how proteins interact with light to determine their structure and concentration.
-
Immunoassays: Detect and quantify specific proteins using antibodies.
Challenges in Therapeutic Protein Analysis
-
Complexity: Proteins have intricate structures and can undergo modifications that affect their function.
-
Heterogeneity: Therapeutic proteins can exist in multiple forms due to variations in glycosylation or other modifications.
-
Low concentrations: Some therapeutic proteins are present at very low levels in biological samples.
Advancements in the Field
-
High-resolution mass spectrometry: Enables more detailed analysis of protein structure and modifications.
-
Advanced chromatography techniques: Improve separation and quantification of proteins.
-
Automation: Increases efficiency and throughput of analytical methods
Antibody Drug Conjugates
Antibody-Drug Conjugates (ADCs): A Deep Dive
ADCs represent a sophisticated class of targeted cancer therapies, combining the precision of antibodies with the potency of cytotoxic drugs. Their development and manufacturing require meticulous attention to detail, necessitating a range of analytical techniques to ensure safety and efficacy.
Components of an ADC
-
Monoclonal Antibody (mAb): A highly specific antibody targeting an antigen (protein) preferentially expressed on cancer cells. Analytical Techniques: ELISA, flow cytometry, and surface plasmon resonance (SPR) are used to assess antibody binding affinity and specificity. Mass spectrometry can confirm antibody sequence and identify any post-translational modifications.
-
Cytotoxic Payload: A potent cell-killing drug, often a microtubule disruptor or DNA-damaging agent. Analytical Techniques: High-performance liquid chromatography (HPLC) and mass spectrometry are crucial for characterizing the payload's purity, identity, and potency.
-
Linker: A chemical bridge connecting the antibody and payload. Linkers are designed for stability in circulation and controlled release within the target cell. Analytical Techniques: Mass spectrometry and NMR spectroscopy help determine linker structure and stability. In vitro release assays assess linker cleavage under various conditions.
Mechanism of Action
-
Target Binding: The ADC binds to the target antigen on the cancer cell surface. Analytical Techniques: SPR and biolayer interferometry (BLI) measure the binding kinetics of the ADC to its target.
-
Internalization: The ADC-antigen complex is internalized into the cell via endocytosis. Analytical Techniques: Confocal microscopy and flow cytometry visualize ADC internalization.
-
Drug Release: The linker is cleaved or degraded, releasing the cytotoxic payload. Analytical Techniques: In vitro and in vivo release assays quantify drug release rates. Mass spectrometry identifies the released payload.
-
Cell Death: The released drug disrupts essential cellular processes, leading to apoptosis (programmed cell death). Analytical Techniques: Cell viability assays and flow cytometry assess the cytotoxic effect of the ADC.
Key Considerations and Analytical Techniques
-
Target Selection: Ensuring the target antigen's specificity to cancer cells is paramount. Analytical Techniques: Immunohistochemistry (IHC) assesses antigen expression in normal and cancerous tissues.
-
Payload Potency and Characterization: The drug's mechanism of action and potency must be well-understood. Analytical Techniques: Cell-based assays determine the drug's IC50 (half-maximal inhibitory concentration).
-
Linker Stability and Cleavage: The linker's properties are critical for ADC performance. Analytical Techniques: In vitro stability studies in various media (e.g., plasma) assess linker integrity.
-
Drug-to-Antibody Ratio (DAR): The average number of drug molecules per antibody impacts efficacy and safety. Analytical Techniques: Hydrophobic interaction chromatography (HIC), size-exclusion chromatography (SEC) coupled with multi-angle light scattering (MALS), and mass spectrometry determine DAR.
-
ADC Aggregation and Stability: Aggregation can affect ADC activity and immunogenicity. Analytical Techniques: SEC-MALS, dynamic light scattering (DLS), and analytical ultracentrifugation (AUC) assess aggregation levels.
Advantages of ADCs
-
Targeted Delivery: Minimizes off-target toxicity.
-
Improved Efficacy: Delivers higher drug concentrations to cancer cells.
-
Treatment of Resistant Cancers: May overcome drug resistance mechanisms.
Challenges and Limitations
-
Off-Target Toxicity: Can occur if the target antigen is expressed on healthy cells.
-
Drug Resistance: Cancer cells may develop resistance to the payload.
-
Complex Manufacturing and Characterization: Requires sophisticated analytical methods.
Applications
ADCs are approved for various cancers, and research is ongoing to expand their applications.
Ongoing Research
Focuses on developing novel antibodies, payloads, and linkers to improve ADC efficacy and safety. Advanced analytical techniques play a crucial role in these advancements.
Intact Protein Analysis - Antibodies
LC-MS Method for Antibody Analysis (General)
This method outlines a general LC-MS approach for antibody analysis. Specific parameters will need optimization based on the antibody and the specific information desired (e.g., intact mass, peptide mapping, post-translational modifications).
I. Sample Preparation:
-
Antibody Source: Purified antibody in a suitable buffer (e.g., PBS, ammonium bicarbonate).
-
Reduction (Optional): If analyzing heavy and light chains separately, reduce disulfide bonds with a reducing agent (e.g., DTT, TCEP) followed by alkylation (e.g., iodoacetamide) to prevent reformation.
-
Digestion (For Peptide Mapping): If peptide mapping is required, digest the antibody with a protease (e.g., trypsin, chymotrypsin, Lys-C) at an appropriate temperature and time. Stop the reaction with an inhibitor.
-
Desalting: Remove salts using C18 ZipTips, SPE cartridges, or other appropriate desalting methods. Crucial for good ionization.
-
Concentration (If Needed): Concentrate the sample if necessary using a vacuum concentrator or other method.
II. Liquid Chromatography (LC):
-
Column:
-
Intact Antibody: Size-exclusion chromatography (SEC) column or reversed-phase LC column (for smaller antibodies or fragments).
-
Peptide Mapping: Reversed-phase C18 column or similar.
-
-
Mobile Phase:
-
Intact Antibody: Aqueous buffer with organic modifier (e.g., acetonitrile) and often a volatile acid (e.g., formic acid) for reversed-phase. SEC uses appropriate buffers.
-
Peptide Mapping: Aqueous buffer with increasing organic modifier (e.g., acetonitrile) and formic acid.
-
-
Flow Rate: Optimized for the column and desired separation (typically 0.2-1 mL/min).
-
Gradient (For Peptide Mapping or Reversed-Phase Intact): A gradient of increasing organic solvent is used for elution. Isocratic for SEC.
-
Injection Volume: Optimized for sensitivity and avoiding column overload.
III. Mass Spectrometry (MS):
-
Ionization: Electrospray ionization (ESI) in positive mode is most common.
-
Mass Analyzer:
-
Intact Antibody: Time-of-flight (TOF), Orbitrap, or Q-TOF are preferred for accurate mass measurement.
-
Peptide Mapping: Triple quadrupole (for targeted analysis), Q-TOF, or Orbitrap.
-
-
Detection:
-
Intact Antibody: Total ion current (TIC) or selected ion monitoring (SIM) for specific charge states.
-
Peptide Mapping: Selected reaction monitoring (SRM) or multiple reaction monitoring (MRM) for targeted peptides. Data-dependent acquisition (DDA) or parallel reaction monitoring (PRM) for comprehensive peptide identification.
-
-
MS/MS (For Peptide Mapping): Collision-induced dissociation (CID), higher-energy collisional dissociation (HCD), or electron transfer dissociation (ETD) for peptide fragmentation and sequencing.
IV. Data Analysis:
-
Software: Specialized proteomics or protein characterization software is required.
-
Intact Antibody: Deconvolution software to determine the intact protein mass.
-
Peptide Mapping: Peptide identification using database searching (e.g., Mascot, Sequest) or de novo sequencing.
-
Post-translational Modifications (PTMs): Identification of PTMs based on mass shifts and fragmentation patterns.
V. Considerations:
-
Calibration: Use appropriate calibration standards for accurate mass measurement.
-
Sensitivity: Optimize sample preparation, LC, and MS parameters for maximum sensitivity.
-
Matrix Effects: Minimize matrix effects from buffers or other components.
-
Reproducibility: Use internal standards and quality control samples to ensure reproducibility.
Disclaimer: This is a general method. Optimization is crucial for each specific antibody and application. Consult with an LC-MS expert for assistance.
Ligand Binding Assay by LC MS (And Native Spray Analysis)
LC-MS Method for Ligand Binding Assay/Native Intact Protein Analysis This method focuses on using LC-MS for studying ligand binding to proteins (including antibodies) or analyzing native, intact proteins.
(Note: there are several high-quality ligan/binding products on the market, not using LC MS.)
I. Sample Preparation:
-
Protein & Ligand: Prepare the protein (antibody or other) and ligand at appropriate concentrations in a compatible buffer.
-
Incubation: Mix the protein and ligand and incubate under conditions that allow for binding (time, temperature, etc.). Establish appropriate controls (protein only, ligand only).
-
Separation of Bound/Unbound (If Necessary):
-
For Ligand Binding: If studying binding affinity, you may need to separate bound from unbound ligand (e.g., using ultrafiltration, size-exclusion chromatography, or other methods). This is not always required for MS-based methods, especially if you are monitoring the complex directly.
-
For Intact Protein: No separation is needed; the protein is analyzed directly.
-
-
Desalting/Buffer Exchange (If Necessary): Remove salts or exchange buffer if required for LC-MS compatibility (e.g., using size exclusion chromatography, buffer exchange columns or spin filters).
II. Liquid Chromatography (LC):
-
Column:
-
Ligand Binding (Complex): Size-exclusion chromatography (SEC) is often preferred to maintain the non-covalent complex. Reversed-phase may be used if the complex is stable enough.
-
Intact Protein: SEC is most common for native protein analysis. Reversed-phase can be used for smaller proteins if appropriate.
-
-
Mobile Phase:
-
Ligand Binding/Intact Protein: Aqueous buffer compatible with the protein and ligand. Volatile buffers (e.g., ammonium acetate, ammonium bicarbonate) are often used for MS. Avoid detergents if possible.
-
-
Flow Rate: Optimized for the column and desired separation.
-
Injection Volume: Adjusted based on sensitivity needs and column capacity.
-
Isocratic or Gradient: Isocratic for SEC; a shallow gradient might be used for some reversed-phase separations.
III. Mass Spectrometry (MS):
-
Ionization: Electrospray ionization (ESI) is the most common. Native ESI conditions may be used for intact protein analysis to preserve non-covalent interactions.
-
Mass Analyzer:
-
Ligand Binding: Time-of-flight (TOF), Orbitrap, or Q-TOF are preferred for accurate mass measurement of the protein-ligand complex.
-
Intact Protein: TOF, Orbitrap, or Q-TOF are preferred.
-
-
Detection:
-
Ligand Binding: Monitor the m/z of the protein-ligand complex and/or the unbound protein and ligand. Deconvolution is usually required.
-
Intact Protein: Monitor the m/z of the protein. Deconvolution is essential.
-
-
MS/MS (If Needed): Collision-induced dissociation (CID), higher-energy collisional dissociation (HCD), or electron transfer dissociation (ETD) can be used for structural information.
IV. Data Analysis:
-
Software: Specialized proteomics or protein characterization software is required for deconvolution and data analysis.
-
Ligand Binding: Determine the stoichiometry of binding, calculate binding affinity (if appropriate), and identify binding sites (if combined with MS/MS).
-
Intact Protein: Determine the intact protein mass and identify any post-translational modifications.
V. Considerations:
-
Non-covalent Interactions: For ligand binding, optimize MS conditions (e.g., source temperature, cone voltage) to preserve non-covalent interactions. Native MS conditions may be required.
-
Deconvolution: Essential for analyzing complex mass spectra of intact proteins and protein-ligand complexes.
-
Sensitivity: Optimize all steps for maximum sensitivity, especially if working with low protein concentrations.
-
Controls: Include appropriate controls (protein only, ligand only) to ensure accurate interpretation of results.
-
Buffer Compatibility: Ensure that the buffer used is compatible with both the protein/ligand and the LC-MS system.
Disclaimer: This is a general method. Optimization is crucial for each specific protein, ligand, and application. Consult with an LC-MS expert for assistance.
Host Cell Protein Analysis - LC MS Based Methods
Host Cell Proteins (HCP) Analysis
Why HCP Analysis Matters
-
Immunogenicity: HCPs can trigger immune responses in patients, leading to adverse effects and potentially reducing drug efficacy.
-
Product Stability: Some HCPs may be enzymes that degrade the antibody or affect its stability.
-
Regulatory Requirements: Regulatory agencies (e.g., FDA, EMA) require strict monitoring and control of HCPs in therapeutic antibodies.
LC-MS-Centric Method for HCP Analysis
This method focuses on using LC-MS/MS for identification and quantification of HCPs.
-
Sample Preparation:
-
Obtain samples of the antibody at various purification stages (e.g., post-protein A, post-ion exchange).
-
Prepare a "mock" sample containing the same components as the antibody sample but without the antibody itself. This serves as a control for matrix effects.
-
Optional Enrichment: If desired, use immunoaffinity purification to enrich for HCPs. This can improve sensitivity.
-
Protein Digestion: Digest proteins (including HCPs) into peptides using a protease (e.g., trypsin).
-
Desalting: Remove salts and other contaminants using C18 ZipTips, SPE cartridges, or other desalting techniques.
-
-
Liquid Chromatography (LC):
-
Column: Reversed-phase C18 column or similar.
-
Mobile Phase: Aqueous buffer (e.g., water with 0.1% formic acid) and organic mobile phase (e.g., acetonitrile with 0.1% formic acid).
-
Gradient: Use a gradient of increasing organic solvent to separate peptides.
-
Flow Rate: Optimize for the column and MS system.
-
-
Mass Spectrometry (MS):
-
Ionization: Electrospray ionization (ESI) in positive ion mode.
-
Mass Analyzer: High-resolution mass spectrometer (e.g., Orbitrap, Q-TOF).
-
Acquisition Mode: Data-dependent acquisition (DDA) or data-independent acquisition (DIA) to acquire MS/MS spectra for peptide identification.
-
-
Data Analysis:
-
Database Searching: Use a protein database (e.g., UniProt) specific to the host cell (e.g., CHO) to identify peptides and proteins.
-
Label-Free Quantification: Use software to quantify HCPs based on peak areas or spectral counting.
-
Data Normalization: Normalize data to account for differences in sample loading and instrument variability.
-
Other Methods for HCP Analysis
-
ELISA (Enzyme-Linked Immunosorbent Assay): Uses antibodies to detect and quantify HCPs.
-
Advantages: Sensitive, high-throughput.
-
Limitations: Requires specific anti-HCP antibodies, may not detect all HCPs.
-
-
2D Gel Electrophoresis (2D-GE) and Western Blotting: Separates proteins by isoelectric point and molecular weight, followed by antibody-based detection.
-
Advantages: Can visualize a wide range of HCPs.
-
Limitations: Time-consuming, less sensitive than ELISA and LC-MS.
-
Important Considerations for LC-MS-Based HCP Analysis
-
Host Cell Database: Use a comprehensive and up-to-date protein database for the host cell.
-
Mock Samples: Include mock samples to assess matrix effects and improve accuracy.
-
Dynamic Range: LC-MS may have challenges with the wide dynamic range of HCPs. Enrichment strategies can help.
-
Data Analysis: Requires specialized software and expertise.
-
Orthogonal Methods: Consider combining LC-MS with ELISA for a more complete picture of HCPs.
Disclaimer: This is a general overview and method. HCP analysis is complex, and specific methods and acceptance criteria will depend on the product, process, and regulatory requirements. Always consult with experts and relevant guidelines.