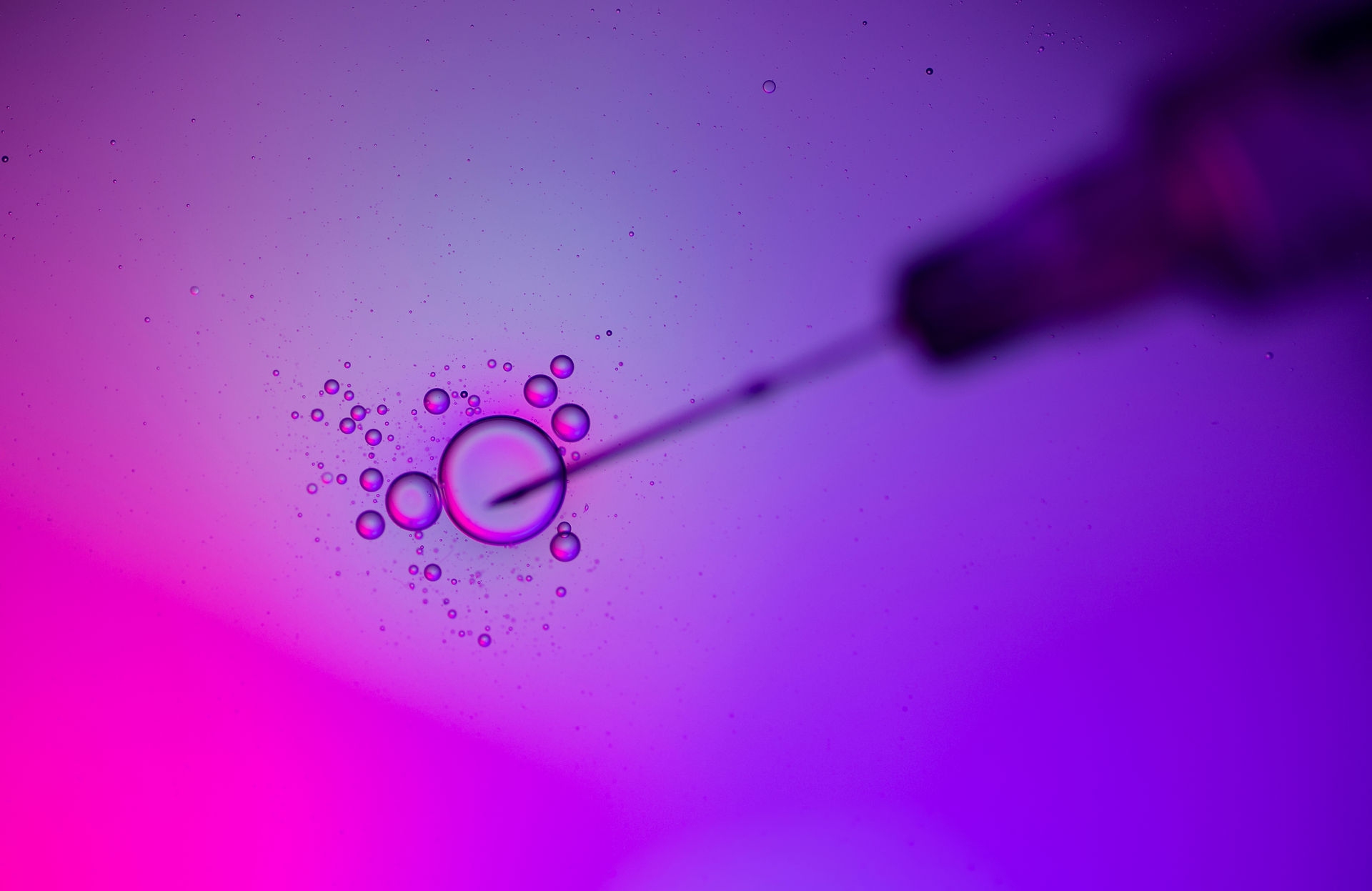
Chromatography Optimization: Achieving Separation Excellence
Example: C18 Information
Example: HILIC Information
Example - Micro Liquid Chromatography
Library - Best Practices for General LC MS - A 101 Presentation
Chromatography Optimization: Achieving Separation Excellence
Overview of Liquid Chromatography - Standard LC, µLC, nLC
What is Gradient Elution?
-
Gradient elution is a technique used in liquid chromatography (LC) where the composition of the mobile phase (solvent) is changed gradually during the separation.
-
This is in contrast to isocratic elution, where the mobile phase composition remains constant throughout the separation.
-
Typically, a gradient starts with a high percentage of a weak solvent (e.g., water in reversed-phase LC) and gradually increases the percentage of a strong solvent (e.g., acetonitrile or methanol in reversed-phase LC).
Why Use Gradient Elution?
-
Improved Separation of Complex Mixtures: Gradients are particularly useful for separating complex mixtures containing compounds with a wide range of polarities.
-
In isocratic elution, early-eluting compounds may be poorly resolved, while late-eluting compounds may have broad peaks and long retention times.
-
Gradients help to resolve early-eluting compounds while also speeding up the elution of late-eluting compounds, resulting in improved overall separation and sharper peaks.
-
-
Reduced Analysis Time: Gradients can significantly shorten analysis times compared to isocratic elution, especially for complex samples.
-
Increased Peak Capacity: Gradient elution can increase the peak capacity (the number of peaks that can be separated) of a chromatographic separation.
-
Enhanced Sensitivity: By focusing the elution of analytes into narrower peaks, gradients can improve sensitivity.
Types of Gradients:
-
Linear Gradient: The percentage of strong solvent increases linearly over time.
-
Step Gradient: The mobile phase composition changes in a series of discrete steps.
-
Curved Gradient: The percentage of strong solvent changes in a non-linear fashion (e.g., concave, convex).
Key Considerations for Gradient Elution:
-
Gradient Steepness: The rate at which the mobile phase composition changes affects the separation. Steeper gradients generally lead to faster separations but may compromise resolution.
-
Initial and Final Mobile Phase Composition: The starting and ending mobile phase compositions should be chosen based on the polarity of the analytes and the stationary phase.
-
Column Equilibration: It's crucial to allow sufficient time for the column to equilibrate with the initial mobile phase composition before starting a gradient.
-
Detector Compatibility: Ensure that your detector is compatible with the solvents used in the gradient.
-
Reproducibility: Careful control of gradient parameters is essential for reproducible separations.
Applications of Gradient Elution:
-
Analysis of complex biological samples (e.g., proteomics, metabolomics)
-
Pharmaceutical analysis (e.g., drug impurities, degradation products)
-
Environmental analysis (e.g., pesticides, pollutants)
-
Food analysis (e.g., additives, contaminants)
By understanding the principles and applications of gradient elution, you can optimize your LC separations for improved resolution, speed, and sensitivity.
Chromatography Optimization: Achieving Separation Excellence
Solvents and Gradients for LC
Liquid Chromatography (LC): An Overview
-
What is Liquid Chromatography?
-
LC is a separation technique used to isolate individual components within a mixture dissolved in a liquid.
-
It works by passing the liquid sample through a column packed with a stationary phase (a solid material with specific chemical properties).
-
Different components in the mixture interact differently with the stationary phase, causing them to move through the column at different speeds and thus separate.
-
-
Why is LC Used?
-
Separation of Complex Mixtures: LC is widely used to separate complex mixtures in various fields, including pharmaceuticals, environmental science, food chemistry, and biological research.
-
Purification: LC can be used to purify compounds of interest by separating them from impurities or other unwanted components.
-
Analysis: LC is often coupled with other analytical techniques, such as mass spectrometry (MS) or ultraviolet-visible (UV-Vis) spectroscopy, to identify and quantify the separated components.
-
Versatility: LC can be used to analyze a wide range of compounds, from small molecules to large biomolecules, by choosing appropriate stationary phases and mobile phases.
-
Components of a Liquid Chromatograph:
-
Pump: Delivers the mobile phase (solvent) through the system at a controlled flow rate.
-
Injector: Introduces the liquid sample into the flowing mobile phase stream.
-
Column: Contains the stationary phase and is where the separation occurs.
-
Detector: Monitors the eluent (the liquid exiting the column) and generates a signal when a component is detected. Common detectors include UV-Vis, fluorescence, and refractive index detectors.
Different Flow Regimes in LC:
-
Standard Flow LC (1 mL/min to 200 µL/min):
-
Description: This is the most common flow regime used in LC. It offers a good balance of separation efficiency, speed, and compatibility with various detectors.
-
Advantages:
-
Well-established and widely used.
-
Compatible with a wide range of LC columns and detectors.
-
Offers good separation efficiency for many applications.
-
-
Disadvantages:
-
Higher solvent consumption compared to lower flow regimes.
-
May not be ideal for very limited sample amounts.
-
-
-
Microflow LC (2 - 20 µL/min):
-
Description: Microflow LC uses lower flow rates than standard flow, resulting in improved sensitivity and reduced solvent consumption.
-
Advantages:
-
Improved sensitivity due to increased analyte concentration in the eluent.
-
Reduced solvent consumption and waste generation.
-
Can be used with smaller sample amounts.
-
-
Disadvantages:
-
Requires specialized microflow LC pumps and columns.
-
Can be more technically challenging than standard flow.
-
-
-
Nanoflow LC (100 nL/min to 750 nL/min):
-
Description: Nanoflow LC uses the lowest flow rates, providing the highest sensitivity and allowing for the analysis of extremely limited sample amounts.
-
Advantages:
-
Highest sensitivity among the flow regimes.
-
Ideal for analyzing very small sample amounts (e.g., single cells, proteomics).
-
Minimal solvent consumption and waste generation.
-
-
Disadvantages:
-
Requires specialized nanoflow LC systems and columns.
-
Most technically challenging flow regime.
-
Can be more susceptible to clogging and other technical issues.
-
-
Key Considerations When Choosing a Flow Regime:
-
Sample amount: Nanoflow is best for very limited samples, while standard flow is suitable for larger quantities.
-
Sensitivity requirements: Nanoflow offers the highest sensitivity, followed by microflow and then standard flow.
-
Detector compatibility: Ensure that your LC detector is compatible with the desired flow regime.
-
Technical expertise: Nanoflow LC requires the highest level of technical expertise.
-
Application: The specific application will also influence the choice of flow regime. For example, proteomics analyses often benefit from nanoflow LC due to the complexity and limited quantity of samples.
Chromatography Optimization: Achieving Separation Excellence
C18 For Reversed Phase LC MS - Most Commonly Used
C18 Material in Liquid Chromatography: A Versatile Stationary Phase
What is C18 Material?
-
C18 material, also known as octadecylsilane (ODS), is a widely used stationary phase in liquid chromatography (LC).
-
It consists of silica particles that have been chemically modified with octadecyl chains (C18 chains), which are long hydrocarbon chains containing 18 carbon atoms.
-
These C18 chains are nonpolar, making C18 a reversed-phase stationary phase. This means that it retains nonpolar compounds more strongly than polar compounds.
Common Applications of C18 Material:
-
Pharmaceutical Analysis: Used to separate and analyze drug compounds, impurities, and metabolites.
-
Environmental Analysis: Used to detect and quantify pollutants, such as pesticides and herbicides, in water and soil samples.
-
Food Analysis: Used to analyze food components, such as vitamins, additives, and contaminants.
-
Bioanalysis: Used to separate and analyze biomolecules, such as peptides, proteins, and oligonucleotides.
Example Types of C18 Chemistries:
C18 phases can be further modified to fine-tune their selectivity and performance. Here are 10 examples of different C18 chemistries:
-
Monomeric C18: The most common type, consisting of a single C18 chain bonded to the silica surface.
-
Polymeric C18: Contains multiple C18 chains bonded to the silica surface, providing higher carbon load and increased retention for nonpolar compounds.
-
Endcapped C18: The silanol groups (Si-OH) on the silica surface are capped with a smaller alkyl group (e.g., trimethylsilyl) to reduce unwanted interactions with polar analytes.
-
Polar-Embedded C18: Contains polar functional groups embedded within the C18 chains, providing unique selectivity for polar and nonpolar compounds.
-
Aqua C18: Designed for improved retention of polar compounds in highly aqueous mobile phases.
-
Phenyl-Hexyl C18: Combines C18 with phenyl groups, offering alternative selectivity for aromatic compounds.
-
C18 with Embedded Polar Groups: Contains polar groups embedded within the C18 chains, providing unique selectivity for polar and nonpolar compounds.
-
Superficially Porous C18: Consists of a solid silica core surrounded by a porous shell, offering improved efficiency and faster separations compared to fully porous particles.
-
High-Strength Silica C18: Made with high-strength silica particles, allowing for higher pressure operation and faster separations.
-
Monodisperse C18: Consists of silica particles with a very narrow size distribution, providing improved efficiency and reproducibility.
Key Considerations When Choosing a C18 Phase:
-
Hydrophobicity: The length and density of the C18 chains affect the hydrophobicity of the phase.
-
Silanol Activity: The presence of residual silanol groups can cause unwanted interactions with polar analytes.
-
Carbon Load: Higher carbon load generally leads to increased retention for nonpolar compounds.
-
Pore Size: The pore size of the silica particles affects the accessibility of analytes to the stationary phase.
-
Particle Size: Smaller particles generally provide higher efficiency and resolution.
-
Endcapping: Endcapping can reduce unwanted interactions with polar analytes.
-
pH Stability: The stability of the C18 phase at different pH values is important for certain applications.
By carefully considering these factors, you can select the most appropriate C18 phase for your specific separation needs.
Chromatography Optimization: Achieving Separation Excellence
HILIC For Normal Phase LC MS - Improved Resolution and Results
Hydrophilic Interaction Liquid Chromatography (HILIC): An Overview
What is HILIC?
-
HILIC is a variant of normal-phase liquid chromatography (NPLC) that is particularly well-suited for separating polar and hydrophilic compounds.
-
It uses a hydrophilic stationary phase (e.g., silica, diol, amide) and a mobile phase consisting of a high percentage of organic solvent (e.g., acetonitrile) with a small amount of water.
-
In HILIC, polar analytes partition into a water-rich layer that forms on the surface of the stationary phase, leading to their retention.
Common Applications of HILIC:
-
Metabolomics: Used to analyze polar metabolites, such as sugars, amino acids, and nucleotides, in biological samples.
-
Pharmaceutical Analysis: Used to separate and analyze polar drug compounds and their metabolites.
-
Environmental Analysis: Used to detect and quantify polar pollutants, such as pesticides and herbicides, in water and soil samples.
-
Food Analysis: Used to analyze polar food components, such as carbohydrates and amino acids.
-
Glycomics: Used to separate and analyze glycans and glycoconjugates.
Example Types of HILIC Stationary Phases:
HILIC offers a diverse range of stationary phases with varying selectivity. Here are 10 examples:
-
Bare Silica: The most basic HILIC phase, offering strong retention for polar compounds.
-
Diol: Contains diol groups (two hydroxyl groups) that provide a more moderate polarity and different selectivity compared to bare silica.
-
Amide: Contains amide groups, offering unique selectivity for hydrogen bond donors and acceptors.
-
Amino: Contains amino groups, providing a different selectivity compared to amide phases.
-
Zwitterionic: Contains both positive and negative charges, offering a wide range of interactions with analytes.
-
Polymeric: Contains a polymer coating on the silica surface, providing different selectivity and stability.
-
Ionic Liquids: Uses ionic liquids as the stationary phase, offering unique selectivity and high thermal stability.
-
Mixed-Mode: Combines HILIC with other retention mechanisms, such as reversed-phase or ion-exchange, to provide broader selectivity.
-
Chiral: Designed for the separation of chiral compounds (molecules that are mirror images of each other).
-
Superficially Porous: Consists of a solid silica core surrounded by a porous shell, offering improved efficiency and faster separations compared to fully porous particles.
Key Considerations When Choosing a HILIC Phase:
-
Polarity: The polarity of the stationary phase affects the retention of polar analytes.
-
Hydrophilicity: The hydrophilicity of the stationary phase influences the water layer formation and analyte partitioning.
-
Charge: Charged phases can provide additional selectivity based on ionic interactions.
-
Functional Groups: Different functional groups offer unique selectivities.
-
Particle Size: Smaller particles generally provide higher efficiency and resolution.
-
pH Stability: The stability of the HILIC phase at different pH values is important for certain applications.
By carefully considering these factors, you can select the most appropriate HILIC phase for your specific separation needs.
Chromatography Optimization: Achieving Separation Excellence
nano LC MS
Nanoflow Liquid Chromatography (nanoLC): An Overview
What is nanoLC?
-
nanoLC is a specialized form of liquid chromatography (LC) that operates at extremely low flow rates, typically in the range of 100 nL/min to 750 nL/min.
-
It utilizes specialized pumps, columns, and fittings designed to handle these very low flow rates.
-
nanoLC is often coupled with mass spectrometry (MS) for high-sensitivity analysis of complex samples.
Why Use nanoLC?
-
Enhanced Sensitivity: The low flow rates in nanoLC result in increased analyte concentration in the eluent, leading to significantly enhanced sensitivity compared to conventional LC. This is crucial for analyzing samples with limited amounts of material or low-abundance analytes.
-
Reduced Sample Consumption: nanoLC requires very small sample volumes, making it ideal for precious or limited samples, such as those encountered in proteomics, single-cell analysis, and biomarker discovery.
-
Improved Ionization Efficiency: The low flow rates in nanoLC are well-suited for electrospray ionization (ESI), a common ionization technique used in MS. This leads to improved ionization efficiency and better signal-to-noise ratios.
-
Compatibility with Mass Spectrometry: nanoLC is often coupled with faster ToF instruments to achieve high sensitivity and detailed characterization of complex samples.
Key Components of a nanoLC System:
-
Nanoflow Pump: Delivers the mobile phase at precise nanoflow rates.
-
NanoLC Column: Typically packed with small-diameter particles (e.g., 1.7 - 3 µm) and has a narrow inner diameter (e.g., 75 - 150 µm) to maintain high separation efficiency at low flow rates.
-
Autosampler: Injects small sample volumes (e.g., nanoliters) with high precision.
-
Connecting Tubing and Fittings: Specialized low-volume tubing and fittings are used to minimize dead volume and maintain chromatographic integrity.
Applications of nanoLC:
-
Proteomics: Identifying and quantifying proteins in complex biological samples, such as cell lysates, tissues, and biofluids.
-
Metabolomics: Analyzing metabolites in biological samples, including identifying isomers and quantifying changes in metabolite levels.
-
Lipidomics: Studying lipids and their roles in biological processes.
-
Biomarker Discovery: Identifying and validating biomarkers for disease diagnosis and prognosis.
-
Single-Cell Analysis: Analyzing the proteome or metabolome of individual cells.
-
Pharmaceutical Analysis: Characterizing drug compounds, impurities, and metabolites.
Advantages of nanoLC:
-
High sensitivity
-
Low sample consumption
-
Improved ionization efficiency
-
Compatibility with high acquisition speed mass-spectrometers
Disadvantages of nanoLC:
-
Technical complexity: Requires specialized equipment and expertise.
-
Susceptibility to clogging: The small-diameter columns and tubing can be prone to clogging.
-
Lower throughput: Analysis times can be longer compared to conventional LC.
Overall, nanoLC is a valuable tool for high-sensitivity analysis of complex samples, particularly in applications where sample amount is limited or analytes are present at low concentrations
Chromatography Optimization: Achieving Separation Excellence
micro LC MS
Microflow Liquid Chromatography (microLC): An Overview
What is microLC?
-
microLC is a form of liquid chromatography (LC) that operates at flow rates intermediate between standard flow LC and nanoflow LC, typically in the range of 2 - 20 µL/min.
-
It utilizes specialized pumps, columns, and fittings designed to handle these reduced flow rates.
-
microLC offers a balance of sensitivity, throughput, and ease of use, making it suitable for a variety of applications.
Why Use microLC?
-
Enhanced Sensitivity: Compared to standard flow LC, microLC offers improved sensitivity due to the increased analyte concentration in the eluent. This is beneficial for analyzing samples with limited material or low-abundance analytes.
-
Reduced Solvent Consumption: microLC consumes less solvent than standard flow LC, leading to reduced costs and less environmental impact.
-
Improved Ionization Efficiency: The lower flow rates in microLC are generally more compatible with electrospray ionization (ESI) mass spectrometry (MS), resulting in improved ionization efficiency and signal-to-noise ratios.
-
Versatility: microLC can be used with a variety of column dimensions and stationary phases, providing flexibility for different applications.
Key Components of a microLC System:
-
Microflow Pump: Delivers the mobile phase at precise microflow rates.
-
MicroLC Column: Typically has a smaller inner diameter (e.g., 0.5 - 1 mm) than standard LC columns, but larger than nanoflow LC columns.
-
Injector: Capable of injecting microliter volumes of sample.
-
Connecting Tubing and Fittings: Uses low-volume tubing and fittings to minimize dead volume and maintain chromatographic performance.
Applications of microLC:
-
Proteomics: Analyzing peptides and proteins in complex biological samples.
-
Metabolomics: Identifying and quantifying metabolites in various matrices.
-
Lipidomics: Characterizing lipids and their roles in biological systems.
-
Pharmaceutical Analysis: Analyzing drug compounds, impurities, and metabolites.
-
Environmental Analysis: Detecting and quantifying pollutants in environmental samples.
Advantages of microLC:
-
Improved sensitivity compared to standard flow LC
-
Reduced solvent consumption
-
Good ionization efficiency for MS
-
Versatility in column choices and applications
-
Less technically demanding than nanoflow LC
Disadvantages of microLC:
-
Lower sensitivity compared to nanoflow LC
-
Requires specialized equipment
-
May not be suitable for extremely limited sample amounts
Overall, microLC offers a valuable compromise between standard flow LC and nanoflow LC, providing enhanced sensitivity and reduced solvent consumption while maintaining versatility and ease of use.